The Neurogenesis Debate
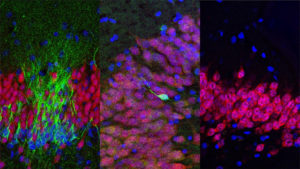
Written by Nicholi Avery
On March 7 2018, a study was published in the highly esteemed journal Nature by an international team of scientists claiming that #neurogenesis starts to rapidly decline in the #human brain as early as 13 years old and becomes undetectable in adults. This rocked the scientific community as there has been a long-established theory that neurogenesis takes place throughout the course of life in the mammalian #brain. Until the 1990s, neurologists were practicing their profession under the doctrine established in the late 19th to early 20th century by the prominent histologist Ramon y Cajal, often referred to as a god of neuroscience;
“Once the development was ended, the founts of growth and regeneration of the axons and dendrites dried up irrevocably. In the adult centers, the nerve paths are something fixed, ended, and immutable. Everything may die, nothing may be regenerated. It is for the science of the future to change, if possible, this harsh decree.”
It wasn’t until later between the 1960s and the 1990s that possible theories for neurogenesis started to develop.
Neurogenesis
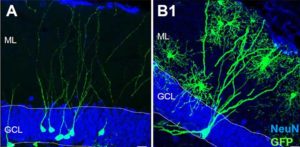
What is neurogenesis exactly?
Neurogenesis is the process by which nerve #cells, known as #neurons, are produced by #neural stem cells (NSC), and it occurs in all animals. This growth is primarily believed to take place in the hippocampus. Despite skeptics, this has been witnessed in a number of vertebrates, and it continues in the mammalian brain throughout life.
Traditionally neurogenesis was believed to only take place during the embryonic and perinatal stages in #mammals. In June 1965 associate professor at MIT Joseph Altman provided ground-breaking research providing histological evidence of postnatal hippocampal neurogenesis in rats. Altman’s research showed that young rats injected with thymidine‐H3 labeled cells declined rapidly with increased age at the time of injection. The researchers write: “The number of labeled cells declined rapidly with increased age at the time of injection. Histological studies showed the presence in young rats of a large germinal matrix of mitotic cells in the ependymal and subependymal layers of the third and lateral ventricles.
The areal extent and cell population of this germinal pool declined rapidly from birth on, with a transient rise with a peak at about 15 days. During this latter period, the number of “undifferentiated” cells near the granular layer of the dentate gyrus showed a rapid rise with a subsequent decline. The decline in the number of “undifferentiated” cells was accompanied by a rise in the number of differentiated granule cells. Cell counts in homologous parts of the dentate gyrus indicated a six‐fold increase in the number of differentiated granule cells from birth to three months.”
Though Altman’s research was published in highly esteemed journals, it was not readily accepted by leaders in the field because as Sapolsky notes in ‘Behave: The Biology of Humans at our Best and Worst’, Altman did not get tenure. Within a few years he lost his funding for his neurogenesis work.
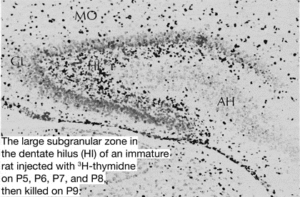
A decade later Michael Kaplan, assistant professor at the University of New Mexico, presented more evidence for neurogenesis utilizing new techniques, also encountering the same problems as Altman did and so, once again, it was rejected by senior figures in the field. The most crushing blow to the neurogenesis debate came from Pasko Rakic who presently works in the Yale School of Medicine Department of Neuroscience in New Haven, Connecticut. Rakic publicly criticized Kaplan’s work and denounced it. This had a devastating effect on Kaplan’s career. He left the field and pursued a career in medicine.
The question as to whether neurogenesis took place in the adult mammalian brain remained an open one and any discussion about neurogenesis was put on hold for another 15 years.
Another unexpected breakthrough in the evidence for neurogenesis took place by a highly esteemed and accomplished neuroscientist named Fernando Nottebohm. A study conducted by Paton JA and Nottebohm FN in 1984 successfully demonstrated the functional integration of new neurons in the adult central nervous system. They demonstrated this by injecting adult canaries with 3H-labeled thymidine, a marker of DNA synthesis. Once the birds matured, it was shown that neurons were generated during or after the 3H-labeled thymidine treatment and subsequently incorporated into functional #neural circuits. The critics were silenced by the quality of Fernando’s work.
While this provided evidence that neurogenesis took place within the adult brain of songbirds, what about mammals?
In 1992 Reynolds BA1 and Weiss S. presented data for the generation of neurons and astrocytes from isolated cells of the adult mammalian central nervous system. They did this by isolating cellular striatum of the adult mouse brain and were influenced to rapidly grow within a controlled experimental setting by epidermal growth factor. Finally the acceptance of neurogenesis started to gain some traction in the field.
Soon after this research other neuroscientists were applying newer and more advanced methods developed by Elizabeth Gould of Princeton and Fred “Rusty” Gage of Salk Institute. They were quickly discovering new neurons in not only songbirds, but mammals too. Even Rakic began to rethink his position.
As neuroscience blossomed neurogenesis was observed not only in mice but other rodents as well. However, many scientists were not only skeptical as to how many new cells were actually made by neurogenesis and how long they actually lived, but also questioned whether neurogenesis took place in other mammals such as primates. Though Rakic was becoming more convinced by the evidence of neurogenesis he remained skeptical if it actually occurred where it really counts, such as the cortex. As time went on the debate as to whether neurogenesis took place in primates and monkeys was settled as evidence showed that it does.
Yet the answer as to whether neurogenesis took place in the human brain remained elusive.
Dr. Eriksson and team from the Department of Clinical Neuroscience, Institute of Neurology, Sahlgrenska University Hospital, Göteborg , Sweden set out to test what many scientists in the field have been wondering ever since neurogenesis was demonstrated to take place in primates. Does neurogenesis take place in the human brain as well?
During the fall of November 1st in 1998 Eriksson and team published the first peer-reviewed article on whether neurogenesis takes place in the mature human brain.
Eriksson obtained human brain tissue that was postmortem from patients who had been treated with the thymidine analog, bromodeoxyuridine (BrdU), which labels DNA during the S phase. In order to show that new neurons they used immunofluorescent labeling for BrdU and for one of the neuronal markers, NeuN, calbindin or neuron specific enolase (NSE), showing that new neurons, as defined by the markers, are generated from dividing progenitor cells in the dentate gyrus of adult humans. The results were conclusive! The team wrote: “we demonstrate that new neurons, as defined by these markers, are generated from dividing progenitor cells in the dentate gyrus of adult humans. Our results further indicate that the human hippocampus retains its ability to generate neurons throughout life.”
This was a huge success for Eriksson. The loss of neurons was thought to be irreversible and is one of the leading causes for brain disease. The discovery of neurogenesis could offer key insight into how we can offset brain related diseases as well as provide treatment. It also gives insight into one of the most amazing things about our brain.
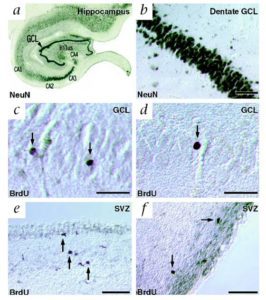
As time went on more evidence was accumulated for neurogenesis taking place in the human brain. Here is a brief timescale of the evidence since Eriksson’s breakthrough research:
1) 2000 Fred “Rusty” Gage PhD showed that neural #stem-cells not only exist in the developing mammalian nervous system but also in the adult nervous system of all mammalian organisms, including humans. Mammalian neural stem cells.
2) 2004 Arturo Alvarez PhD demonstrated that the adult mammalian brain retains neural #stem cells that continually generate new neurons within two restricted regions: the subventricular zone (SVZ) of the lateral ventricle and the dentate gyrus subgranular zone (SGZ) of the hippocampus. Alvarez also discovered the common themes that begin to define adult neurogenic niches. Maintaining Germinal Niches in the Adult Brain.
3) 2006 Pierre-Marie Liedo PhD discovered that the #plasticity of the brain morphological and physiological changes are possible at many levels, including that of the entire cell, even in the face of a varying environments. Adult neurogenesis and functional plasticity in neuronal circuits.
4) 2004 Arturo Alvarez PhD demonstrated that the adult mammalian brain retains neural stem cells that continually generate new neurons within two restricted regions: the subventricular zone (SVZ) of the lateral ventricle and the dentate gyrus subgranular zone (SGZ) of the hippocampus. Alvarez also discovered the common themes that begin to define adult neurogenic niches. How wide spread Is adult neurogenesis in mammals?
5) 2008 Xin Duan, PhD demonstrated the sequential steps of adult neurogenesis, ranging from neural precursor proliferation to synaptic integration of newborn neurons. Development of neural stem cell in the adult brain.
6) 2010 Wei Deng, James B. Aimone & Fred H. Gage explored how the adult hippocampal neurogenesis affects learning and memory making important discoveries. New neurons and new memories: how does adult hippocampal neurogenesis affect learning and memory?
While the evidence for neurogenesis is compelling, the newly published study mentioned above casts doubt on the long established evidence based upon neurogenesis. The study was led by PhD Robert Sapolsky’s graduate student Shawn Sorrells and a team of international scientists.
They document what much of the earlier evidence for neurogenesis seemed to indicate, that the birth of new neuron takes place in the early development of mammalian brain. However Sorrells and team claim to have demonstrated that a defined population of progenitor cells does not coalesce in the subgranular zone during human fetal or postnatal development. They also state that they found that the number of proliferating progenitors and young neurons in the dentate gyrus declines sharply during the first year of life and only a few isolated young neurons are observed by 7 and 13 years of age.
This is a far cry from the decades worth of research presented in favor of neurogenesis.
Does Neurogenesis exist in the human brain? I reached out to world renowned neuroendocrinologist Robert Sapolsky inquiring about this latest study. In an email Sapolsky assured me that they were extremely careful and that he completely believes the data of the study. Sapolsky also stated that the key to this study in comparison to his own work is that it involves human brains (and is the most thorough study of adult human neurogenesis ever done), whereas everything he has done is with rodents. Though I highly respect Doctor Sapolsky, other neuroscientists, are skeptical of such a report as it is the first of its kind.
Dr. Sandrine Thuret supports the long established data of neurogenesis and she believes that the failed replication of one experiment for neurogenesis is not convincing enough to dismiss decades worth of research. Dr. Sandrine Thuret is Principal Investigator & Lecturer in Neural Stem Cell Research at the prestigious King’s college London.
Dr. Saul Villeda in a recent paper demonstrated that the tet2 molecule found in the blood of younger mice plays a vital role in the development of new neurons. Villeda believes that neurogenesis is a signpost of regeneration in the brain, but thinks ultimately that it is changes to the neurons themselves preventing synapse loss, and boosting plasticity that will improve cognition. Villeda is hopeful that a similar molecule may help birth new neurons in the human brain as well.
Does neurogenesis take place in the adult mammalian brain?
One thing is for certain, to break free of old belief systems is not as simple as being exposed to evidence or even proof that the beliefs are wrong.
Even in the scientific community, which prides itself on reason and rationality, we see a long history of proof being ignored for years, decades, even generations. It has been said that science progresses “funeral by funeral” as we wait for the “old guard” to die off for new facts to be accepted.
Scientists “knew” that surgeons using disinfectants and washing their hands before attending to childbirth would prevent infections and save lives. The doctors ignored the evidence and refused to change their practices of going straight from autopsies to surgeries. It took years for this practice of handwashing to finally be accepted.
It requires a willingness to accept the truth after we have discovered it.
Examples like this are numerous and extend to every field of human endeavor.
The debate in the scientific community on neurogenesis demonstrates this all too well.
On April 5th, 2018, the latest study of neurogenesis was published in Cell claiming to once again have demonstrated the growth of new neurons in the hippocampal region of the adult human brain. This particular study is important because the researchers utilized the method of stereology—the gold standard of neurogenesis studies in animals—to count immature and mature neurons in the hippocampi of healthy humans. Stereology can determine the number of individual cell types in tissue, even when the sample has been sliced into sections. Lead researcher Maura Boldrini et al. state: “We found similar numbers of intermediate neural progenitors and thousands of immature neurons in the DG, comparable numbers of glia and mature granule neurons, and equivalent DG volume across ages.
Nevertheless, older individuals have less angiogenesis and neuroplasticity and a smaller quiescent progenitor pool in anterior-mid DG, with no changes in posterior DG. Thus, healthy older subjects without cognitive impairment, neuropsychiatric disease, or treatment display preserved neurogenesis. It is possible that ongoing hippocampal neurogenesis sustains human-specific cognitive function throughout life and that declines may be linked to compromised cognitive-emotional resilience.”
The understanding of neurogenesis is important as it would give scientists data as to how the brain can repair itself which could lead to breakthrough research that could treat brain related diseases or damage such as Alzheimer’s disease.
I shared my article with Dr. Sorrells and he requested that I share the critique of the study in Cell:
In our view, the new study’s exclusive reliance on labeling cellular proteins associated with new neurons is not sufficient evidence to conclude that the cells being observed really are new neurons. Based on the representative images they present, the cells they call new neurons in the adult hippocampus are very different in shape and appearance from what would be considered a young neuron in other species, or what we have observed in humans in young children.
This is why in our study we also performed careful analysis of cell shape and structure using light and electron microscopes, which revealed that similarly labeled cells in our own adult brain samples proved to be neither young neurons nor neural progenitors, but rather non-neuronal glial cells expressing similar molecular markers.
We also have technical concerns about some of the key protein labeling performed in this study. Based on the images presented in the paper, some of these labels appear to be dominated by optical contamination (“bleedthrough”) from other stains used in the same experiments, further calling into question the results overall.
Finally, we also question the assertion by Boldrini et al that the subgranular zone (SGZ), the key site of adult neurogenesis in the rodent brain, is present in their adult human brain samples. None of their histology actually shows a layer of small, frequently proliferating cells and their blood vessel staining in figure 4 fails to show the dense plexus of blood vessels that characterizes the SGZ in rodents and is considered a key structure for neurogenesis in the adult mammalian brain. This is consistent with our own observation that in humans the SGZ never coalesces as a supportive site for adult neurogenesis in the dentate gyrus.
Given the unclear nature of the cells presented, the lack of a clear SGZ, and the developmental trajectory that we describe in our study, with new neurons clearly present in children but not in adults, we think that the simplest interpretation is that neurogenesis in the adult human dentate gyrus is absent or extremely rare. It boils down to interpretation of equivocal cells which we took extra steps to characterize extensively and showed not to be new neurons as they first appeared.
Cipriani2018 (1)
In the future we may need to look at how we can artificially stimulate neurogenesis within the brain or creating artificial neurons.
Resources and citations:
“Human Hippocampal Neurogenesis Persists Throughout Aging.”
Cell Volume 22, Issue 4, p589–599.e5, 5 April 2018
Full Abstract
“Human hippocampal neurogenesis drops sharply in children to undetectable levels in adults.”
Nature volume555, pages377–381 (15 March 2018)
Full Abstract
“Neurons generated in the adult brain are recruited into functional circuits.”
Science 07 Sep 1984: Vol. 225, Issue 4666, pp. 1046–1048
Full Abstract
“De novo generation of neuronal cells from the adult mouse brain.”
PNAS September 15, 1992. 89 (18) 8591–8595
Full Abstract
“Neurogenesis in the adult human hippocampus.”
Nature Medicine volume4, pages1313–1317 (1998)
Full Abstract
“Generation of neurons and astrocytes from isolated cells of the adult mammalian central nervous system.”
Science 27 Mar 1992: Vol. 255, Issue 5052, pp. 1707–1710
Full Abstract
“Autoradiographic and histological evidence of postnatal hippocampal neurogenesis in rats.”
Wiley Online Library First published: June 1965
Full Abstract