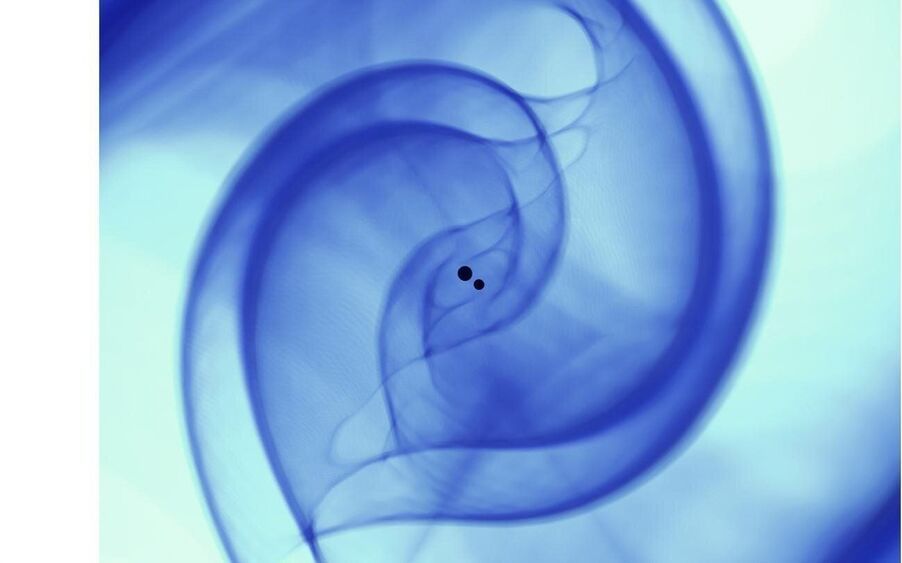
When gravitational waves were first detected in 2015 by the advanced Laser Interferometer Gravitational-Wave Observatory (LIGO), they sent a ripple through the scientific community, as they confirmed another of Einstein’s theories and marked the birth of gravitational wave astronomy. Five years later, numerous gravitational wave sources have been detected, including the first observation of two colliding neutron stars in gravitational and electromagnetic waves.
As LIGO and its international partners continue to upgrade their detectors’ sensitivity to gravitational waves, they will be able to probe a larger volume of the universe, thereby making the detection of gravitational wave sources a daily occurrence. This discovery deluge will launch the era of precision astronomy that takes into consideration extrasolar messenger phenomena, including electromagnetic radiation, gravitational waves, neutrinos and cosmic rays. Realizing this goal, however, will require a radical re-thinking of existing methods used to search for and find gravitational waves.
Recently, computational scientist and lead for translational artificial intelligence (AI) Eliu Huerta of the U.S. Department of Energy’s (DOE) Argonne National Laboratory, in conjunction with collaborators from Argonne, the University of Chicago, the University of Illinois at Urbana-Champaign, NVIDIA and IBM, has developed a new production-scale AI framework that allows for accelerated, scalable and reproducible detection of gravitational waves.