When it comes to singularities, black holes are only half the story. White holes — regions of space where nothing can enter — are returning to the experimental spotlight.
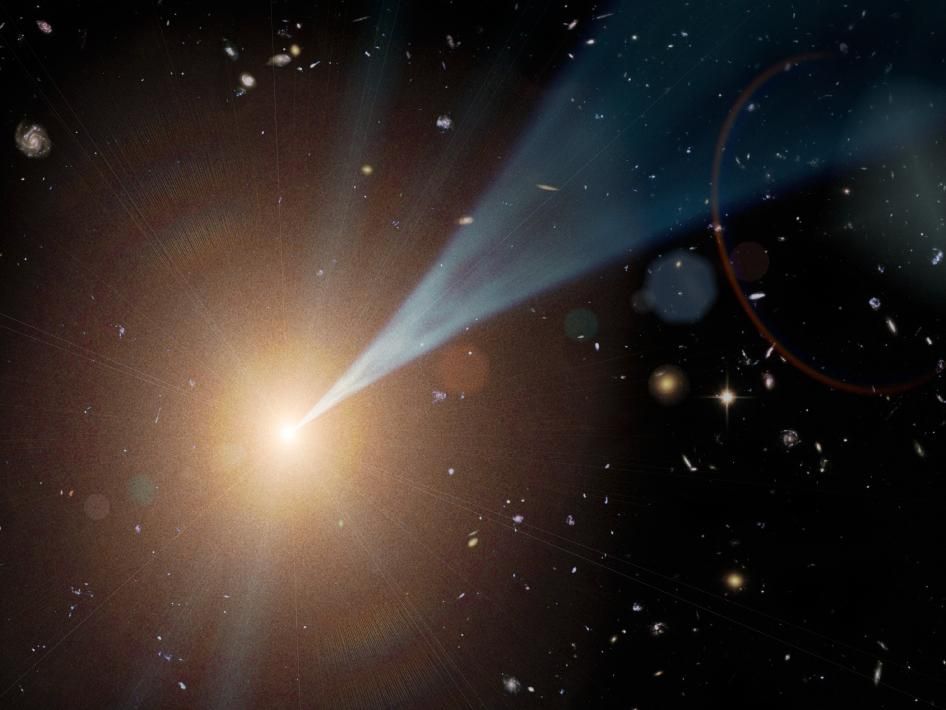
How did matter gain the edge over antimatter in the early universe? Maybe, just maybe, neutrinos.
The Super-Kamiokande Neutrino Observatory, located more than 3,000 feet below Mount Ikeno near the city of Hida, Japan. Credit… Kamioka Observatory, Institute for Cosmic Ray Research, University of Tokyo.
O,.o possible higgs field containment device could stop the rupture and other ways to destroy the root of the problem too.
New data from NASA’s Hubble Space Telescope details what may be the most powerful phenomena in the universe: the “quasar tsunami,” a cosmic storm of such terrifying proportions that it can tear apart an entire galaxy.
“No other phenomena carries more mechanical energy,” said principal investigator Nahum Arav of Virginia Tech in a statement. “The winds are pushing hundreds of solar masses of material each year. The amount of mechanical energy that these outflows carry is up to several hundreds of times higher than the luminosity of the entire Milky Way galaxy.”
Black Hole Death
Arav and colleagues described the devastating phenomena in a series of six papers published in The Astrophysical Journal Supplements.
Scientists have made a major breakthrough that could help us understand the origin of our universe, they say.
Researchers have discovered hints of a difference between the behaviour of neutronos and antineutrinos. That, in turn, could help demonstrate why there is so much matter relative to antimatter in the universe – and, in turn, how everything that surrounds us came to be.
:oooo.
Past cosmological and astrophysical observations suggest that over one quarter of the universe’s energy density is made up of a non-conventional type of matter known as dark matter. This type of matter is believed to be composed of particles that do not absorb, emit or reflect light, and thus cannot be observed directly using conventional detection methods.
Researchers worldwide have carried out studies aimed at detecting dark matter in the universe, yet so far, none of them has been successful. Even the preferred candidate for dark matter, weakly interacting massive particles (WIMPs), have not yet been observed experimentally.
The China Dark Matter Experiment (CDEX) collaboration, a large team of researchers at Tsinghua University and other universities in China, has recently conducted a search for a different possible dark matter candidate known as the dark photon. While the search was unsuccessful, their paper, published in Physical Review Letters, identifies new constraints on a dark photon parameter that could inform future studies.
Could be made into a generator of some kind :3.
One of the strangest effects to arise from the quantum nature of the universe is the Casimir force. This pushes two parallel conducting plates together when they are just a few dozen nanometres apart.
At these kinds of scales, the Casimir force can dominate and engineers are well aware of its unwanted effects. One reason why microelectromechanical machines have never reached their original promise is the stiction that Casimir forces can generate.
On the other hand, many engineers hope to exploit the Casimir force. Various theoretical models predict that the force should be repulsive between objects of certain shapes, a phenomenon that could prevent stiction.
A neutron star is the dead husk of a star more massive than the sun, but not large enough to become a black hole upon its demise. These stars are between 10 and 29 solar masses during their active lifetime. When they exhaust their nuclear fuel and go supernova, all that’s left is the ultra-dense collapsed core. We call that a neutron star.
The wild physics inside a neutron star are down to the incredible mass packed into such a small space. A neutron star might have twice the mass of our sun packed into an object just a few miles across. The crush of gravity contorts and squeezes neutrons into unusual configurations, based on the models developed by scientists studying neutron stars.
Scientists currently believe that neutron stars have layers characterized by different configurations of distorted neutron matter. For whatever reason, researchers have decided to name the various structures after pasta. Near the surface there’s gnocchi, which are round bubble-like neutrons. Go a bit deeper, and the pressure forces neutrons into long tubes called spaghetti. Go further down, and you have sheets of neutrons called lasagna. That’s just the start of the Italian-inspired interior of neutron stars.