200+ user-developed early quantum applications on D-Wave systems, including airline scheduling, election modeling, quantum chemistry simulation, automotive design, preventative healthcare, logistics, and much more.
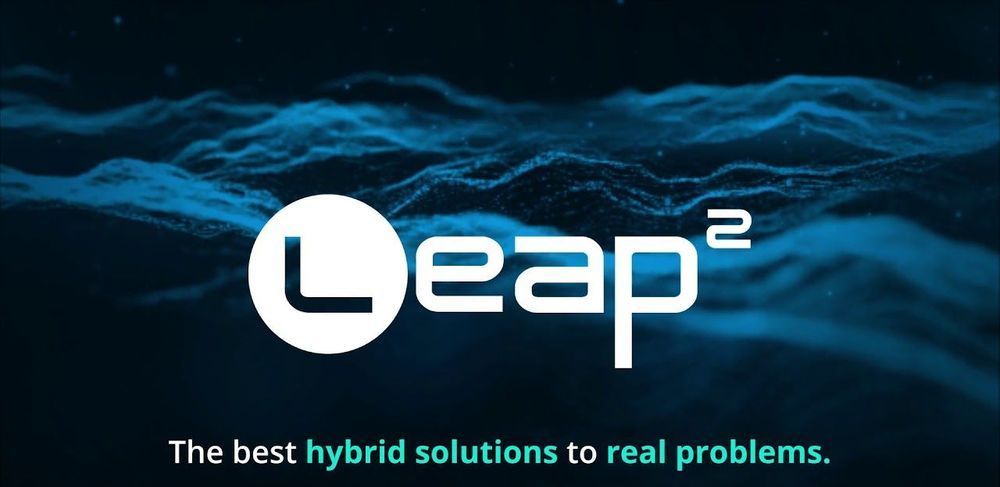
200+ user-developed early quantum applications on D-Wave systems, including airline scheduling, election modeling, quantum chemistry simulation, automotive design, preventative healthcare, logistics, and much more.
A Nobel Prize winning biology professor has provided a bit of good news amidst the coronavirus gloom; the US may see a downturn in new cases sooner than some models have predicted.
Michael Levitt, a Stanford University biology professor and a 2013 Nobel Prize winner in chemistry, said his models predict the virus is not likely to dwindle on for months or years and – most importantly – is not likely to cause millions of deaths.
Mr Levitt previously predicted – correctly – when China would experience and endure the worst of its coronavirus crisis.
The race toward the first practical quantum computer is in full stride. Companies, countries, collaborators, and competitors worldwide are vying for quantum supremacy. Google says it’s already there. But what does that mean? How will the world know when it’s been achieved?
Using classical computers, computational scientists at PNNL have set a mark that a quantum system would need to surpass to establish quantum supremacy in the realm of chemistry.
That’s because the fastest classical computers available today are getting better and better at simulating what a quantum computer will eventually be expected to do. To prove itself in the real world, a quantum computer will need to be able to outdo what a fast supercomputer can do. And that’s where the PNNL-led team have set a benchmark for quantum computers to beat.
Quantum computing isn’t yet far enough along that it could have helped curb the spread of this coronavirus outbreak. But this emerging field of computing will almost certainly help scientists and researchers confront future crises.
“Can we compress the rate at which we discover, for example, a treatment or an approach to this?” asks Dario Gil, the director of IBM Research. “The goal is to do everything that we are doing today in terms of discovery of materials, chemistry, things like that, (in) factors of 10 times better, 100 times better,”
And that, he says, “could be game-changing.”
The team compared germ-free (sterile) mice and mice with normal microbes. They used a laboratory technique called mass spectrometry to characterize the non-living molecules in every mouse organ. They identified as many molecules as possible by comparing them to reference structures in the GNPS database, a crowdsourced mass spectrometry repository developed by Dorrestein and collaborators. They also determined which living microbes co-locate with these molecules by sequencing a specific genetic region that acts as a barcode for bacterial types.
In total, they analyzed 768 samples from 96 sites of 29 different organs from four germ-free mice and four mice with normal microbes. The result was a map of all of the molecules found throughout the body of a normal mouse with microbes, and a map of molecules throughout a mouse without microbes.
A comparison of the maps revealed that as much as 70 percent of a mouse’s gut chemistry is determined by its gut microbiome. Even in distant organs, such as the uterus or the brain, approximately 20 percent of molecules were different in the mice with gut microbes.
Scientists at the U.S. Department of Energy’s Lawrence Berkeley National Laboratory (Berkeley Lab) have made a surprising discovery that could help explain our risk for developing chronic diseases or cancers as we get older, and how our food decomposes over time.
What’s more, their findings, which were reported recently in the Proceedings of the National Academy of Sciences (PNAS), point to an unexpected link between the ozone chemistry in our atmosphere and our cells’ hardwired ability to ward off disease.
“The beauty of nature is that it often decides to use similar chemistries throughout a system, but we never thought that we would find a common link between atmospheric chemistry, and the chemistry of our bodies and food,” said Kevin Wilson, the deputy director of Berkeley Lab’s Chemical Sciences Division who led the study. “Our study is the first to explore another chemical pathway that might affect how well the cells in our bodies — and even our food — can respond to oxidative stress, such as pollution, over time.”
“[Einstein] dreamt that he was riding a sled down a steep, snowy slope and, as he approached the speed of light in his dream, the colors all blended into one. He spent much of his career, inspired by that dream, thinking about what happens at the speed of light.”
By Tara MacIsaac, Epoch Times
In Beyond Science, Epoch Times explores research and accounts related to phenomena and theories that challenge our current knowledge. We delve into ideas that stimulate the imagination and open up new possibilities.
1. Dmitri Mendeleev, Periodic Table
Dmitri Mendeleev (1834–1907) wanted to organize the 65 known elements somehow. He knew there was a pattern to be discerned, and it had something to do with atomic weight, but the pattern remained elusive. Then, Mendeleev later reported, “In a dream I saw a table where all the elements fell into place as required. Awakening, I immediately wrote it down on a piece of paper.” Mendeleev’s words were quoted in “On the Question of Scientific Creativity,” by Russian chemist B.M. Kedrov.
For the first time, researchers have used light to control the shape of nanoparticles and create micron-size hollow shells from crystals of cuprous oxide (copper and oxygen). Such particles could have future applications as a low-cost catalyst to help pull excess carbon dioxide from the air, a way to improve microscopic imaging and more, says Bryce Sadtler, a chemist at Washington University in St. Louis and senior author of a study on the new method, published last October in Chemistry of Materials.
Hollowed-out microcrystals could lock away carbon.
In a wild galaxy over half a billion light-years away, astronomers have detected molecular oxygen. It’s only the third such detection ever outside the Solar System — and the first outside the Milky Way.
Oxygen is the third most abundant element in the Universe, behind hydrogen (naturally) and helium. So its chemistry and abundance in interstellar clouds are important for understanding the role of molecular gas in galaxies.
Astronomers have searched for oxygen again and again, using millimetre astronomy, which detects the radio wavelengths emitted by molecules; and spectroscopy, which analyses the spectrum to look for wavelengths absorbed or emitted by specific molecules.