Advanced materials that seem like they come from Star Trek are becoming reality today.
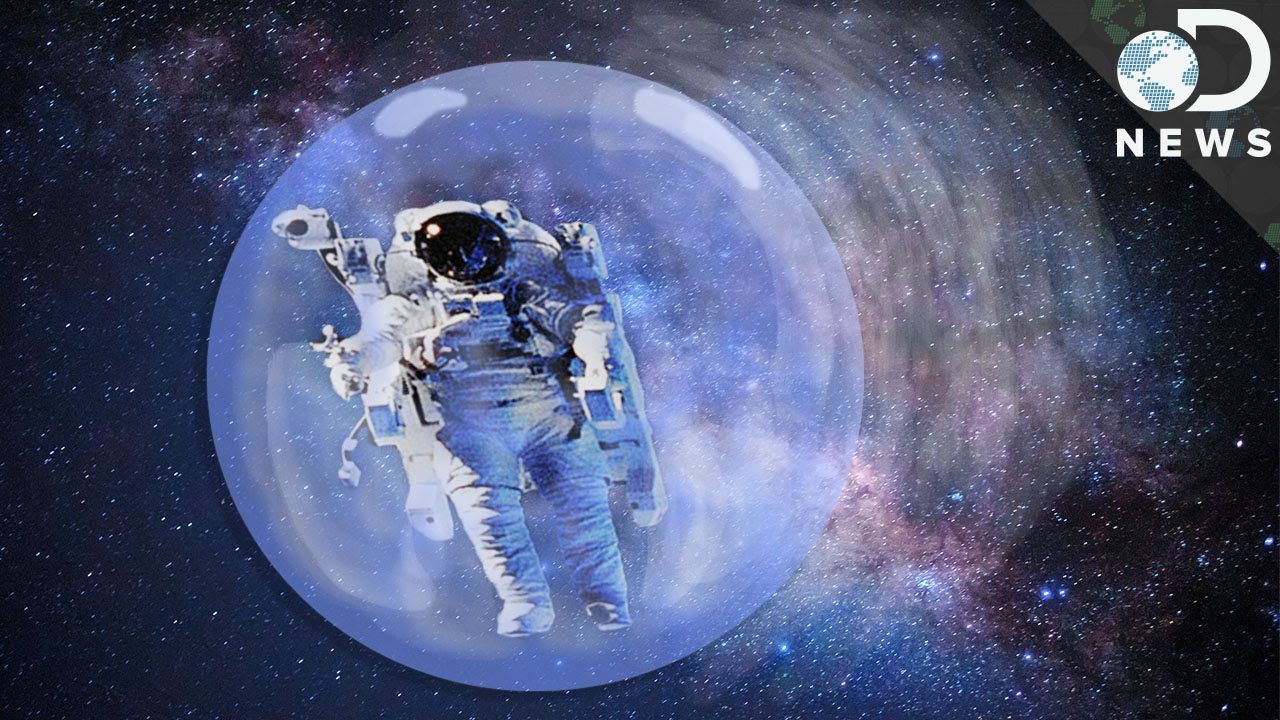
Google unveiled software aimed at making it easier for scientists to use the quantum computers in a move designed to give a boost to the nascent industry.
The software, which is open-source and free to use, could be used by chemists and material scientists to adapt algorithms and equations to run on quantum computers. It comes at a time when Google, IBM, Intel Corp., Microsoft Corp. and D-Wave Systems Inc. are all pushing to create quantum computers that can be used for commercial applications.
The relationship between the mind and the brain is a mystery that is central to how we understand our very existence as sentient beings. Some say the mind is strictly a function of the brain — consciousness is the product of firing neurons. But some strive to scientifically understand the existence of a mind independent of, or at least to some degree separate from, the brain.
The peer-reviewed scientific journal NeuroQuantology brings together neuroscience and quantum physics — an interface that some scientists have used to explore this fundamental relationship between mind and brain.
An article published in the September 2017 edition of NeuroQuantology reviews and expands upon the current theories of consciousness that arise from this meeting of neuroscience and quantum physics.
A team of researchers with Università degli Studi di Padova and the Matera Laser Ranging Observatory in Italy has conducted experiments that add credence to John Wheeler’s quantum theory thought experiment. In their paper published on the open access site Science Advances, the group describes their experiment and what they believe it showed.
The nature of light has proven to be one of the more difficult problems facing physicists. Nearly a century ago, experiments showed that light behaved like both a particle and a wave, but subsequent experiments seemed to show that light behaved differently depending on how it was tested, and weirdly, seemed to know how the researchers were testing it, changing its behavior as a result.
Back in the late 1970s, physicist Johan Wheeler tossed around a thought experiment in which he asked what would happen if tests allowed researchers to change parameters after a photon was fired, but before it had reached a sensor for testing—would it somehow alter its behavior mid-course? He also considered the possibilities as light from a distant quasar made its way through space, being lensed by gravity. Was it possible that the light could somehow choose to behave as a wave or a particle depending on what scientists here on Earth did in trying to measure it? In this new effort, the team in Italy set out to demonstrate the ideas that Wheeler had proposed—but instead of measuring light from a quasar, they measured light bounced from a satellite back to Earth.
D-Wave system shows quantum computers can learn to detect particle signatures in mountains of data, but doesn’t outpace conventional methods — yet.
(Phys.org)—Ever since the early days of quantum mechanics, the decay dynamics of unstable quantum systems has been thought to follow an exponential decay law, just like the one used to describe radioactive decay and many other natural processes. The exponential law in the quantum domain was originally proposed by George Gamow and later developed by Eugene Wigner and Victor Weisskopf. According to this law, when given a sample of unstable atoms, the number of those that are likely to decay during a brief period of time is proportional to the number of atoms present.
In the years since then, however, physicists have found that deviations from the exponential law can occur in unstable quantum systems, but only in those that are isolated from the external environment. This is because isolated systems are free from environmental decoherence, which makes it possible for the quantum decay products to reconstruct themselves back into their initial, pre-decayed states. Consequently, the decay is initially slower than that predicted by the exponential law, and in the later stages, the decay often exhibits a power-law behavior. Researchers have previously shown that this nonexponential decay can be harnessed for quantum control.
Now in a new study, physicists have theoretically shown that quantum decay processes can deviate from the exponential decay law not only when the system is isolated, but even when it is in contact with the external environment. The results suggest that an unstable quantum system can decay and subsequently return to its original state, even in the presence of environmental decoherence.
One of the secrets to making tiny laser devices such as opthalmic surgery scalpels work even more efficiently is the use of tiny semiconductor particles, called quantum dots. In new research at Los Alamos National Laboratory’s Nanotech Team, the ~nanometer-sized dots are being doctored, or “doped,” with additional electrons, a treatment that nudges the dots ever closer to producing the desired laser light with less stimulation and energy loss.
“When we properly tailor the compositional profile within the particles during their fabrication, and then inject two or more electrons in each dot, they become more able to emit laser light. Importantly, they require considerably less power to initiate the lasing action,” said Victor Klimov, leader of the Nanotech team.
In order to force a material to emit laser light one has to work toward a “population inversion,” that is, making the number of electrons in a higher-energy electronic state exceed the number that are in a lower-energy state. To achieve this condition normally, one applies an external stimulus (optical or electrical) of a certain power, which should exceed a critical value termed the “optical-gain threshold.” In a recent paradigm-changing advance, Los Alamos researchers demonstrated that by adding extra electrons into their specially designed quantum dots, they can reduce this threshold to virtually zero.
In a classical thermodynamic system, heat current flows from the hotter body to the colder one, or electricity from the higher voltage to the lower one. The same thing happens in quantum systems, but this state can be changed, and the flow of energy and particles can be reversed if a quantum observer is inserted into the system.
This is the main result obtained by the group led by Professor Ángel Rubio of the UPV/EHU and of the Max Planck Institute PMSD, together with collaborators at the BCCMS centre in Bremen. Their study has been published in npj Quantum Materials.
In macroscopic objects such as a current of water, observing the current does not affect the flow of the water and, in accordance with the laws of classical thermodynamics, this flow would take place from the upper to the lower part of the system. However, in quantum systems, “the process of observation changes the state of the system, and this makes it more likely that the current will be made to flow in one direction or another,” says Ángel Rubio, a professor with the Hamburg-based Max Planck Institute for the Structure and Dynamics of Matter.
Quantum theory predicts that a vast number of atoms can be entangled and intertwined by a very strong quantum relationship, even in a macroscopic structure. Until now, however, experimental evidence has been mostly lacking, although recent advances have shown the entanglement of 2,900 atoms. Scientists at the University of Geneva (UNIGE), Switzerland, recently reengineered their data processing, demonstrating that 16 million atoms were entangled in a one-centimetre crystal. They have published their results in Nature Communications.
The laws of quantum physics allow immediately detecting when emitted signals are intercepted by a third party. This property is crucial for data protection, especially in the encryption industry, which can now guarantee that customers will be aware of any interception of their messages. These signals also need to be able to travel long distances using special relay devices known as quantum repeaters—crystals enriched with rare earth atoms and cooled to 270 degrees below zero (barely three degrees above absolute zero), whose atoms are entangled and unified by a very strong quantum relationship. When a photon penetrates this small crystal block, entanglement is created between the billions of atoms it traverses. This is explicitly predicted by the theory, and it is exactly what happens as the crystal re-emits a single photon without reading the information it has received.
It is relatively easy to entangle two particles: Splitting a photon, for example, generates two entangled photons that have identical properties and behaviours. Florian Fröwis, a researcher in the applied physics group in UNIGE’s science faculty, says, “But it’s impossible to directly observe the process of entanglement between several million atoms since the mass of data you need to collect and analyse is so huge.”