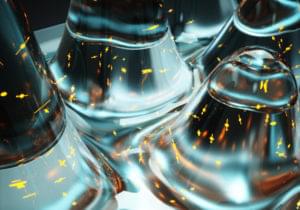
Oct. 13 2021 — In 1,998 researchers including Mark Kubinec of UC Berkeley performed one of the first simple quantum computations using individual molecules. They used pulses of radio waves to flip the spins of two nuclei in a molecule, with each spin’s “up” or “down” orientation storing information in the way that a “0” or “1” state stores information in a classical data bit. In those early days of quantum computers, the combined orientation of the two nuclei – that is, the molecule’s quantum state – could only be preserved for brief periods in specially tuned environments. In other words, the system quickly lost its coherence. Control over quantum coherence is the missing step to building scalable quantum computers.
Now, researchers are developing new pathways to create and protect quantum coherence. Doing so will enable exquisitely sensitive measurement and information processing devices that function at ambient or even extreme conditions. In 2,018 Joel Moore, a senior faculty scientist at Lawrence Berkeley National Laboratory (Berkeley Lab) and professor at UC Berkeley, secured funds from the Department of Energy to create and lead an Energy Frontier Research Center (EFRC) – called the Center for Novel Pathways to Quantum Coherence in Materials (NPQC) – to further those efforts. “The EFRCs are an important tool for DOE to enable focused inter-institutional collaborations to make rapid progress on forefront science problems that are beyond the scope of individual investigators,” said Moore.
Through the NPQC, scientists from Berkeley Lab, UC Berkeley, UC Santa Barbara, Argonne National Laboratory, and Columbia University are leading the way to understand and manipulate coherence in a variety of solid-state systems. Their threefold approach focuses on developing novel platforms for quantum sensing; designing two-dimensional materials that host complex quantum states; and exploring ways to precisely control a material’s electronic and magnetic properties via quantum processes. The solution to these problems lies within the materials science community. Developing the ability to manipulate coherence in realistic environments requires in-depth understanding of materials that could provide alternate quantum bit (or “qubit”), sensing, or optical technologies.