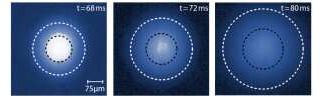
Many phenomena of the natural world evidence symmetries in their dynamic evolution which help researchers to better understand a system’s inner mechanism. In quantum physics, however, these symmetries are not always achieved. In laboratory experiments with ultracold lithium atoms, researchers from the Center for Quantum Dynamics at Heidelberg University have proven for the first time the theoretically predicted deviation from classical symmetry. Their results were published in the journal Science.
“In the world of classical physics, the energy of an ideal gas rises proportionally with the pressure applied. This is a direct consequence of scale symmetry, and the same relation is true in every scale invariant system. In the world of quantum mechanics, however, the interactions between the quantum particles can become so strong that this classical scale symmetry no longer applies,” explains Associate Professor Dr. Tilman Enss from the Institute for Theoretical Physics. His research group collaborated with Professor Dr. Selim Jochim’s group at the Institute for Physics.
In their experiments, the researchers studied the behaviour of an ultracold, superfluid gas of lithium atoms. When the gas is moved out of its equilibrium state, it starts to repeatedly expand and contract in a “breathing” motion. Unlike classical particles, these quantum particles can bind into pairs and, as a result, the superfluid becomes stiffer the more it is compressed. The group headed by primary authors Dr. Puneet Murthy and Dr. Nicolo Defenu—colleagues of Prof. Jochim and Dr. Enss—observed this deviation from classical scale symmetry and thereby directly verified the quantum nature of this system. The researchers report that this effect gives a better insight into the behaviour of systems with similar properties such as graphene or superconductors, which have no electrical resistance when they are cooled below a certain critical temperature.