Intel introducing the ‘Horse Ridge’ to help enable Quantum Computers that are commercially viable, compared to the current models.
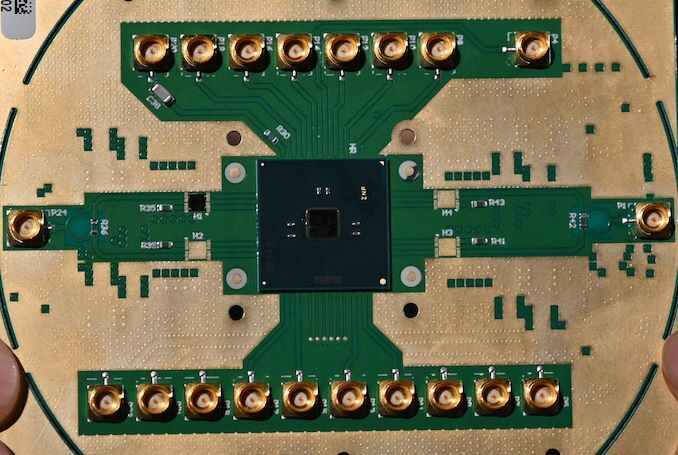
The accelerator-on-a-chip demonstrated in Science is just a prototype, but Vuckovic said its design and fabrication techniques can be scaled up to deliver particle beams accelerated enough to perform cutting-edge experiments in chemistry, materials science and biological discovery that don’t require the power of a massive accelerator.
“The largest accelerators are like powerful telescopes. There are only a few in the world and scientists must come to places like SLAC to use them,” Vuckovic said. “We want to miniaturize accelerator technology in a way that makes it a more accessible research tool.”
Team members liken their approach to the way that computing evolved from the mainframe to the smaller but still useful PC. Accelerator-on-a-chip technology could also lead to new cancer radiation therapies, said physicist Robert Byer, a co-author of the Science paper. Again, it’s a matter of size.
Today, medical X-ray machines fill a room and deliver a beam of radiation that’s tough to focus on tumors, requiring patients to wear lead shields to minimize collateral damage.
On a hillside above Stanford University, the SLAC National Accelerator Laboratory operates a scientific instrument nearly 2 miles long. In this giant accelerator, a stream of electrons flows through a vacuum pipe, as bursts of microwave radiation nudge the particles ever-faster forward until their velocity approaches the speed of light, creating a powerful beam that scientists from around the world use to probe the atomic and molecular structures of inorganic and biological materials.
Now, for the first time, scientists at Stanford and SLAC have created a silicon chip that can accelerate electrons—albeit at a fraction of the velocity of that massive instrument—using an infrared laser to deliver, in less than a hair’s width, the sort of energy boost that takes microwaves many feet.
Writing in the Jan. 3 issue of Science, a team led by electrical engineer Jelena Vuckovic explained how they carved a nanoscale channel out of silicon, sealed it in a vacuum and sent electrons through this cavity while pulses of infrared light—to which silicon is as transparent as glass is to visible light—were transmitted by the channel walls to speed the electrons along.
Quantum computers, quantum cryptography and quantum (insert name here) are often in the news these days. Articles about them inevitably refer to entanglement, a property of quantum physics that makes all these magical devices possible.
Einstein called entanglement “spooky action at a distance,” a name that has stuck and become increasingly popular. Beyond just building better quantum computers, understanding and harnessing entanglement is also useful in other ways.
For example, it can be used to make more accurate measurements of gravitational waves, and to better understand the properties of exotic materials. It also subtly shows up in other places: I have been studying how atoms bumping into each other become entangled, to understand how this affects the accuracy of atomic clocks.
Scientists have uncovered a new kind of electrical process in the human brain that could play a key role in the unique way our brains compute.
Our brains are computers that work using a system of connected brain cells, called neurons, that exchange information using chemical and electric signals called action potentials. Researchers have discovered that certain cells in the human cortex, the outer layer of the brain, transmit signals in a way not seen in corresponding rodent cells. This process might be important to better understanding our unique brains and to improving programs that are based on a model of the human brain.
By Donna Lu
A computer made from strands of DNA in a test tube can calculate the square root of numbers up to 900.
Chunlei Guo at the University of Rochester in New York state and colleagues developed a computer that uses 32 strands of DNA to store and process information. It can calculate the square root of square numbers 1, 4, 9, 16, 25 and so on up to 900.
https://youtu.be/axSnW-ygU5g
Happy New Years!
Here is a little nostalgia and irony all rolled into one. Enjoy.
As 2019 winds to a close, the journey towards fully realised quantum computing continues: physicists have been able to demonstrate quantum teleportation between two computer chips for the first time.
Put simply, this breakthrough means that information was passed between the chips not by physical electronic connections, but through quantum entanglement – by linking two particles across a gap using the principles of quantum physics.
We don’t yet understand everything about quantum entanglement (it’s the same phenomenon Albert Einstein famously called “spooky action”), but being able to use it to send information between computer chips is significant, even if so far we’re confined to a tightly controlled lab environment.
Integrated optics provides a versatile platform for quantum information processing and transceiving with photons1,2,3,4,5,6,7,8. The implementation of quantum protocols requires the capability to generate multiple high-quality single photons and process photons with multiple high-fidelity operators9,10,11. However, previous experimental demonstrations were faced by major challenges in realizing sufficiently high-quality multi-photon sources and multi-qubit operators in a single integrated system4,5,6,7,8, and fully chip-based implementations of multi-qubit quantum tasks remain a significant challenge1,2,3. Here, we report the demonstration of chip-to-chip quantum teleportation and genuine multipartite entanglement, the core functionalities in quantum technologies, on silicon-photonic circuitry. Four single photons with high purity and indistinguishablity are produced in an array of microresonator sources, without requiring any spectral filtering. Up to four qubits are processed in a reprogrammable linear-optic quantum circuit that facilitates Bell projection and fusion operation. The generation, processing, transceiving and measurement of multi-photon multi-qubit states are all achieved in micrometre-scale silicon chips, fabricated by the complementary metal–oxide–semiconductor process. Our work lays the groundwork for large-scale integrated photonic quantum technologies for communications and computations.