San Jose State’s Claire Komives is testing an antivenom inspired by opossum biochemistry against various snake species to prevent deaths in the developing world.
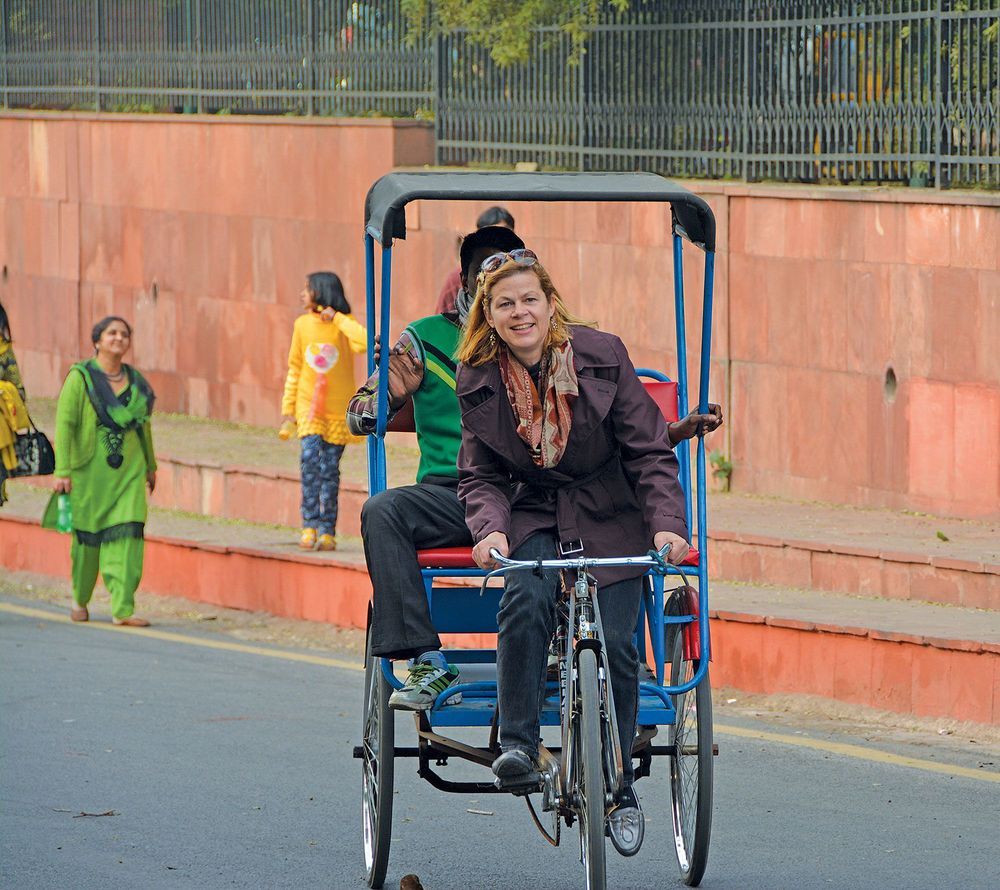
Magnetically separating waste particles makes it possible to reclaim a variety of raw materials from waste. Using a magnetic fluid, a waste flow can be separated into multiple segments in a single step. Researchers from Utrecht and Nijmegen have now succeeded in creating a magnetic fluid that remains stable in extremely strong magnetic fields, which makes it possible to separate materials with a high density, such as electronic components. The results have recently been published in The Journal of Physical Chemistry Letters.
Magnetic density separation
When you drop a stone and a wooden ball into a basin of water, the stone will sink while the ball floats on the surface. This is because the two objects have different densities: the stone is more dense than the water, while the wood is less dense. That principle is also used in magnetic density separation (MDS), except that instead of using water—which has a fixed density—it uses a magnetic fluid with an effective density that can change in relation to its distance from a magnet: it has a higher apparent density at less distance to the magnet. As a result, waste particles of different densities float at different depths in the fluid.
The latest AI algorithms are probing the evolution of galaxies, calculating quantum wave functions, discovering new chemical compounds and more. Is there anything that scientists do that can’t be automated?
The researchers used powerful laser flashes to irradiate thin, films of crystalline materials. These laser pulses drove crystal electrons into a fast wiggling motion. As the electrons bounced off with the surrounding electrons, they emitted radiation in the extreme ultraviolet part of the spectrum. By analyzing the properties of this radiation, the researchers composed pictures that illustrate how the electron cloud is distributed among atoms in the crystal lattice of solids with a resolution of a few tens of picometers which is a billionth of a millimeter.
The experiments pave the way towards developing a new class of laser-based microscopes that could allow physicists, chemists, and material scientists to peer into the details of the microcosm with unprecedented resolution and to deeply understand and eventually control the chemical and the electronic properties of materials.
For decades scientists have used flashes of laser light to understand the inner workings of the microcosm. Such lasers flashes can now track ultrafast microscopic processes inside solids. Still they cannot spatially resolve electrons, that is, to see how electrons occupy the minute space among atoms in crystals, and how they form the chemical bonds that hold atoms together. The reason is long known. It was discovered by Abbe more than a century back. Visible light can only discern objects commensurable in size to its wavelength which is approximately few hundreds of nanometers. But to see electrons, the microscopes have to increase their magnification power by a few thousand times.
California (CNN) (07/17/20) — Scientists have discovered a type of bacteria that eats and gets its calories from metal, after suspecting they exist for more than a hundred years but never proving it.
Now microbiologists from the California Institute of Technology (or Caltech) accidentally discovered the bacteria after performing unrelated experiments using a chalk-like type of manganese, a commonly found chemical element.
Dr. Jared Leadbetter, professor of environmental microbiology at Caltech in Pasadena, left a glass jar covered with the substance to soak in tap water in his office sink, and left the vessel for several months when he went to work off campus.
To calculate the most stable atomic configuration, as well as estimate its hardness, the team relied on a computational method called density functional theory (DFT). DFT has been successfully used throughout chemistry and solid-state physics to predict the structure and properties of materials. Keeping track of the quantum states of all the electrons in a sample, and their interactions, is usually an intractable task. Instead, DFT uses an approximation that focuses on the final density of electrons in space orbiting the atoms. This simplifies the calculation to make it suitable for computers, while still providing very precise results.
Based on these calculations, the scientists found that the Young’s modulus, a measure of hardness, for pentadiamond is predicted to be almost 1700 GPa – compared with about 1200 GPa for conventional diamond.
“Not only is pentadiamond harder than conventional diamond, its density is much lower, equal to that of graphite,” explains co-author Professor Mina Maruyama.
(CNN) — Scientists have discovered a type of bacteria that eats and gets its calories from metal, after suspecting they exist for more than a hundred years but never proving it.
Now microbiologists from the California Institute of Technology (or Caltech) accidentally discovered the bacteria after performing unrelated experiments using a chalk-like type of manganese, a commonly found chemical element.
(CNN)Scientists have discovered a type of bacteria that eats and gets its calories from metal, after suspecting they exist for more than a hundred years but never proving it.
New research shows that one of the heaviest known elements can be manipulated to a greater degree than previously thought, potentially paving the way for new strategies to recycle nuclear fuel and better long-term storage of radioactive elements.
An international team of researchers has demonstrated how curium—element 96 in the periodic table and one of the last that can be seen with the naked eye—responds to the application of high pressure created by squeezing a sample between two diamonds.
Led by Florida State University Professor Thomas Albrecht-Schmitt and collaborators at the University at Buffalo and Aachen University, the team found that the behavior of curium’s outer electrons—which influence its ability to bond with other elements—can be altered by shortening the distance between it and surrounding lighter atoms. The findings are published in the journal Nature.
No industry will be spared.
The pharmaceutical business is perhaps the only industry on the planet, where to get the product from idea to market the company needs to spend about a decade, several billion dollars, and there is about 90% chance of failure. It is very different from the IT business, where only the paranoid survive but a business where executives need to plan decades ahead and execute. So when the revolution in artificial intelligence fueled by credible advances in deep learning hit in 2013–2014, the pharmaceutical industry executives got interested but did not immediately jump on the bandwagon. Many pharmaceutical companies started investing heavily in internal data science R&D but without a coordinated strategy it looked more like re-branding exercise with the many heads of data science, digital, and AI in one organization and often in one department. And while some of the pharmaceutical companies invested in AI startups no sizable acquisitions were made to date. Most discussions with AI startups started with “show me a clinical asset in Phase III where you identified a target and generated a molecule using AI?” or “how are you different from a myriad of other AI startups?” often coming from the newly-minted heads of data science strategy who, in theory, need to know the market.
However, some of the pharmaceutical companies managed to demonstrate very impressive results in the individual segments of drug discovery and development. For example, around 2018 AstraZeneca started publishing in generative chemistry and by 2019 published several impressive papers that were noticed by the community. Several other pharmaceutical companies demonstrated impressive internal modules and Eli Lilly built an impressive AI-powered robotics lab in cooperation with a startup.
However, it was not possible to get a comprehensive overview and comparison of the major pharmaceutical companies that claimed to be doing AI research and utilizing big data in preclinical and clinical development until now. On June 15th, one article titled “The upside of being a digital pharma player” got accepted and quietly went online in a reputable peer-reviewed industry journal Drug Discovery Today. I got notified about the article by Google Scholar because it referenced several of our papers. I was about to discard the article as just another industry perspective but then I looked at the author list and saw a group of heavy-hitting academics, industry executives, and consultants: Alexander Schuhmacher from Reutlingen University, Alexander Gatto from Sony, Markus Hinder from Novartis, Michael Kuss from PricewaterhouseCoopers, and Oliver Gassmann from University of St. Gallen.
Choi and other researchers have also tried to use lithium-ion battery electrodes to pull lithium directly from seawater and brines without the need for first evaporating the water. Those electrodes consist of sandwichlike layered materials designed to trap and hold lithium ions as a battery charges. In seawater, a negative electrical voltage applied to a lithium-grabbing electrode pulls lithium ions into the electrode. But it also pulls in sodium, a chemically similar element that is about 100,000 times more abundant in seawater than lithium. If the two elements push their way into the electrode at the same rate, sodium almost completely crowds out the lithium.
Lithium is prized for rechargeables because it stores more energy by weight than other battery materials. Manufacturers use more than 160,000 tons of the material every year, a number expected to grow nearly 10-fold over the next decade. But lithium supplies are limited and concentrated in a handful of countries, where the metal is either mined or extracted from briny water.
Lithium’s scarcity has raised concerns that future shortages could cause battery prices to skyrocket and stymie the growth of electric vehicles and other lithium-dependent technologies such as Tesla Powerwalls, stationary batteries often used to store rooftop solar power.
Seawater could come to the rescue. The world’s oceans contain an estimated 180 billion tons of lithium. But it’s dilute, present at roughly 0.2 parts per million. Researchers have devised numerous filters and membranes to try to selectively extract lithium from seawater. But those efforts rely on evaporating away much of the water to concentrate the lithium, which requires extensive land use and time. To date such efforts have not proved economical.