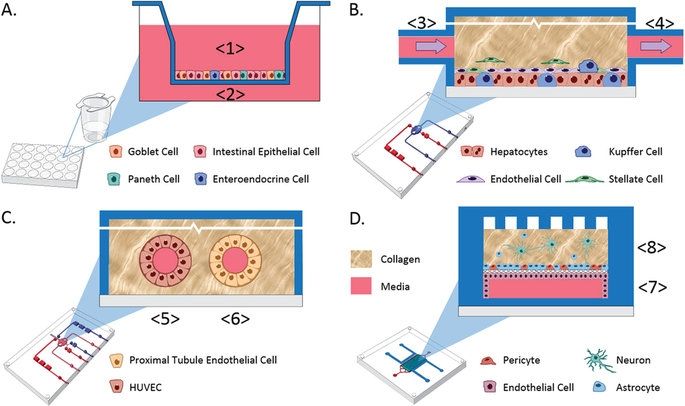
This is definitely a share that is interesting to many studying synthetic organs and their acceptance into the human body as well as the work occurring on Quantum biology as well.
The goal of in vitro and in vivo toxicity testing is to identify compounds that would predict adverse reactions in humans. Olson et al. found that only 70% of human toxicity was predicted from animal testing. Currently we rely on traditional toxicity testing in animals, a 1930’s methodology that is now challenged due to questionable relevance to human risk, high cost, ethical concerns, and throughput that is too limited for the nearly 80,000 industrial chemicals not yet tested for safety. Additionally, testing usually extrapolates acute, high dose animal results to chronic, low dose human exposures, thereby risking rejection or limiting the use of drugs, industrial chemicals or consumer products. Moreover, the ability of lab animal target organ toxicity to predict dose-limiting toxicity in the corresponding human organ varies widely, from a low of 30% for human cutaneous toxicity, to 50–60% for human hepatotoxicity, to a high of 90% for hematological drug toxicity. Animal drug efficacy models are also notoriously discordant. In an analysis of six drugs to treat head injury, hemorrhage, acute ischemic stroke, neonatal respiratory distress syndrome, and osteoporosis, it was found that efficacy was similar in animals and humans for three drugs but was dissimilar for another three. In oncology drug development, animal models often over-predict anti-tumor efficacy in humans3,4. Examples such as these highlight the need to continue research into methods that reduce the dependence on laboratory animals for toxicity testing of environmental chemicals, determine efficacy and toxicity in drug development, serve as a mimic of human diseases, and provide patient-specific guidance in the emerging field of precision medicine.
Recent advances in bioengineered materials, microfluidic technology, and the availability of human primary, immortalized, and induced pluripotent stem cell (iPSC)-derived cells are enabling development of human microphysiological systems (MPS), sometimes called “organs-on-a-chip” or “human-on-a-chip,” that use multiple organ-specific human cells to recapitulate many functional and structural properties of a human organ. It is now generally accepted and supported by data that cellular responses to drugs in most human organs are more accurately approximated in 3D cell cultures than in traditional static 2D cell cultures5,6. Microfluidic perfusion further improves model performance by providing a flow of nutrients and oxygen and the removal of waste products from the cell cultures. Physiologically relevant flow increases oxygen consumption, Krebs cycle activity and secretion of synthesized proteins, and decreases expression of the hypoxia HIF1 gene. Flow also improves the absorption and metabolism of compounds like benzo[a]pyrene6,8,9. The large number of recent publications reviewing organ MPS models indicates a high degree of interest by industrial and academic researchers, granting agencies and other stakeholders10,11,12,13. In addition to the stand-alone MPS, investigators are linking MPS to study organ-organ functional interactions, efficacy, PK and toxicology14,15,16,17,18.
An obvious approach to linking organs is direct coupling of the media stream outflow from one organ into the inflow of the next by use of tubing or a connecting channel. Some limitations to this approach include the requirement for a common medium, difficulty in reducing metabolic wastes to the next organ, organ-specific flow rates and adequate oxygenation of all modules in the system19. These requirements are most easily addressed when the linked organ modules are designed and developed at the same time and in the same laboratory, but even when the organ modules are co-developed, the proper scaling between organ modules is a significant design and calculation challenge. Although organ modules can be sized using allometric scaling20, the resulting functional capacity of the individual organ models may not scale the same.
Read more