A roundup of news from SC21: With Frontier still not online, still no official Exascale systems in the Top500.
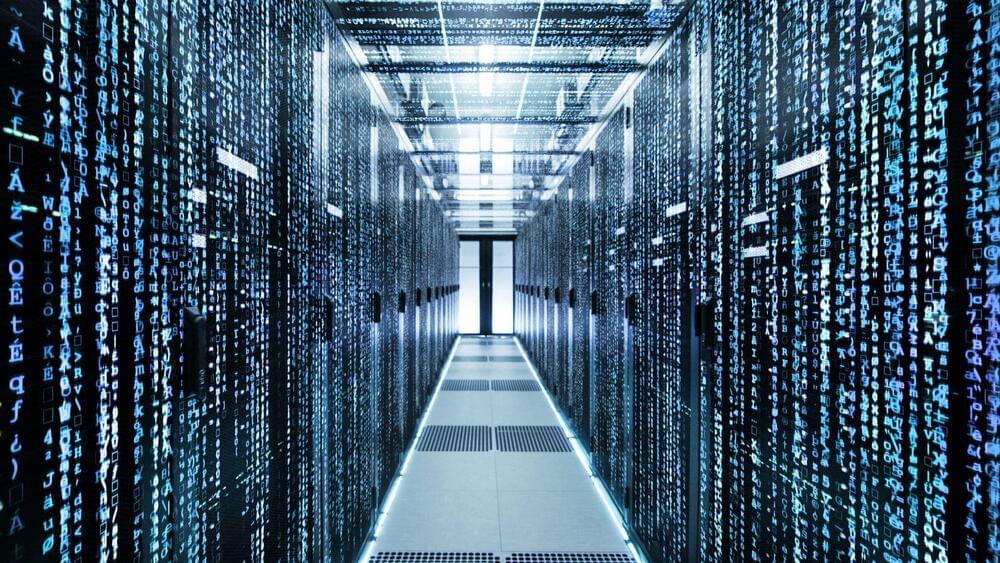
Friends Lunch with a Member.
Topic: Negative Energy, Quantum Information and Causality.
Speaker: Adam Levine.
Date: November 19, 2021
Einstein’s equations of gravity show that too much negative energy can lead to causality violations and causal paradoxes such as the so-called “grandfather paradox. In quantum mechanics, however, negative energies can arise from intrinsically quantum effects, such as the Casimir effect. Thus, it is not clear that gravity and quantum mechanics can be self-consistently combined. In this talk, Levine will discuss modern advances in understanding the connection between energy and causality in gravity and how quantum gravity avoids obvious paradoxes. He will also explore how this line of thought leads to new insights in quantum field theory, which governs particle physics.
As a physicist, Adam Levine’s research aims to understand the structure of entanglement in quantum field theories and quantum gravity through use of techniques from the study of conformal field theories, as well as quantum information theory and AdS/CFT. With support from the National Science Foundation, Adam is a long term Member in the School of Natural Sciences. He received his Ph.D. from University of California, Berkeley (2019), was a Graduate Fellow at the Kavli Institute for Theoretical Physics (2018), a National Defense Science and Engineering Graduate Fellow (2017−2020), and received the Jeffrey Willick Memorial Award for Outstanding Scholarship in Astrophysics from Stanford University (2015).
Researchers prepare ‘new type of matter’ to conduct classic wave-particle duality experiment.
The iconic quantum double-slit experiment, which reveals how matter can behave like waves that displays interference and superposition, has for the first time been demonstrated with individual molecules as the slits.
Richard Feynman once said that the double-slit experiment reveals the central puzzles of quantum mechanics, putting us ‘up against the paradoxes and mysteries and peculiarities of nature’.
Richard Zare, Nandini Mukherjee and their co-workers at Stanford University, US, have now shown that when helium atoms collide with deuterium molecules (D2) in quantum superposition of states, the scattering can take two different paths that interfere with one another. The researchers reveal the interference by looking at its effects on the scattered D2 molecules, which lose rotational energy in the collision.
The ability to precisely control and change properties of a photon, including polarization, position in space, and arrival time, gave rise to a wide range of communication technologies we use today, including the Internet. The next generation of photonic technologies, such as photonic quantum networks and computers, will require even more control over the properties of a photon.
One of the hardest properties to change is a photon’s color, otherwise known as its frequency, because changing the frequency of a photon means changing its energy.
Today, most frequency shifters are either too inefficient, losing a lot of light in the conversion process, or they can’t convert light in the gigahertz range, which is where the most important frequencies for communications, computing, and other applications are found.
Well beyond Descartes and his mind-body duality, new questions have emerged that are as exciting as they are nebulous: Does quantum physics play a role in how the brain works? Or, more profoundly, is the mind, viewed as a collection of possible brain states, sustained by quantum effects? Or can it all be treated using classical physics?
There is nothing better than mixing two great mysteries to produce an even bigger one.
The first 256-qubit quantum computer has been announced by startup company QuEra, founded by MIT and Harvard scientists.
QuEra Computing Inc. – a new Boston, Massachusetts-based company – has emerged from stealth mode with $17 million in funding and has completed the assembly of a 256-qubit device. Its funders include Japanese e-commerce giant Rakuten, Day One Ventures, Frontiers Capital, and the leading tech investors Serguei Beloussov and Paul Maritz. The company recently received a DARPA award, and has already generated $11 million in revenue.
QuEra Computing recently achieved ground-breaking research on neutral atoms, developed at Harvard University and the Massachusetts Institute of Technology, which is being used as the basis for a highly scalable, programmable quantum computer solution. The QuEra team is aiming to build the world’s most powerful quantum computers to take on computational tasks that are currently deemed impossibly hard.
Physicists have created a new ultra-thin, two-layer material with quantum properties that normally require rare earth compounds. This material, which is relatively easy to make and does not contain rare earth metals, could provide a new platform for quantum computing and advance research into unconventional superconductivity and quantum criticality.
The researchers showed that by starting from seemingly common materials, a radically new quantum state of matter can appear. The discovery emerged from their efforts to create a quantum spin liquid which they could use to investigate emergent quantum phenomena such as gauge theory. This involves fabricating a single layer of atomically thin tantalum disulphide, but the process also creates islands that consist of two layers.
When the team examined these islands, they found that interactions between the two layers induced a phenomenon known as the Kondo effect, leading to a macroscopically entangled state of matter producing a heavy-fermion system.
And that’s where physicists are getting stuck.
Zooming in to that hidden center involves virtual particles — quantum fluctuations that subtly influence each interaction’s outcome. The fleeting existence of the quark pair above, like many virtual events, is represented by a Feynman diagram with a closed “loop.” Loops confound physicists — they’re black boxes that introduce additional layers of infinite scenarios. To tally the possibilities implied by a loop, theorists must turn to a summing operation known as an integral. These integrals take on monstrous proportions in multi-loop Feynman diagrams, which come into play as researchers march down the line and fold in more complicated virtual interactions.
Physicists have algorithms to compute the probabilities of no-loop and one-loop scenarios, but many two-loop collisions bring computers to their knees. This imposes a ceiling on predictive precision — and on how well physicists can understand what quantum theory says.
Over the past several decades, researchers have moved from using electric currents to manipulating light waves in the near-infrared range for telecommunications applications such as high-speed 5G networks, biosensors on a chip, and driverless cars. This research area, known as integrated photonics, is fast evolving and investigators are now exploring the shorter—visible—wavelength range to develop a broad variety of emerging applications. These include chip-scale LIDAR (light detection and ranging), AR/VR/MR (augmented/virtual/mixed reality) goggles, holographic displays, quantum information processing chips, and implantable optogenetic probes in the brain.
The one device critical to all these applications in the visible range is an optical phase modulator, which controls the phase of a light wave, similar to how the phase of radio waves is modulated in wireless computer networks. With a phase modulator, researchers can build an on-chip optical switch that channels light into different waveguide ports. With a large network of these optical switches, researchers could create sophisticated integrated optical systems that could control light propagating on a tiny chip or light emission from the chip.
But phase modulators in the visible range are very hard to make: there are no materials that are transparent enough in the visible spectrum while also providing large tunability, either through thermo-optical or electro-optical effects. Currently, the two most suitable materials are silicon nitride and lithium niobate. While both are highly transparent in the visible range, neither one provides very much tunability. Visible-spectrum phase modulators based on these materials are thus not only large but also power-hungry: the length of individual waveguide-based modulators ranges from hundreds of microns to several mm and a single modulator consumes tens of mW for phase tuning. Researchers trying to achieve large-scale integration—embedding thousands of devices on a single microchip—have, up to now, been stymied by these bulky, energy-consuming devices.