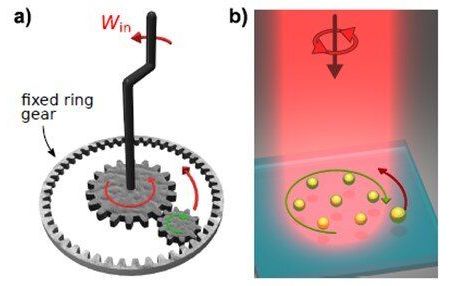
“In previous work, the researchers discovered that when optical matter is exposed to circularly polarized light, it rotates as a rigid body in the direction opposite the polarization rotation. In other words, when the incident light rotates one way the optical matter array responds by spinning the other. This is a manifestation of “negative torque”. The researchers speculated that a machine could be developed based on this new phenomenon.
In the new work, the researchers created an optical matter machine that operates much like a mechanical machine based on interlocking gears. In such machines, when one gear is turned, a smaller interlocking gear will spin in the opposite direction. The optical matter machine uses circularly polarized light from a laser to create a nanoparticle array that acts like the larger gear by spinning in the optical field. This “optical matter gear” converts the circularly polarized light into orbital, or angular, momentum that influences a nearby probe particle to orbit the nanoparticle array (the gear) in the opposite direction.”
Researchers have developed a tiny new machine that converts laser light into work. These optically powered machines self-assemble and could be used for nanoscale manipulation of tiny cargo for applications such as nanofluidics and particle sorting.
“Our work addresses a long-standing goal in the nanoscience community to create self-assembling nanoscale machines that can perform work in conventional environments such as room temperature liquids,” said research team leader Norbert F. Scherer from the University of Chicago.
Scherer and colleagues describe the new nanomachines in Optica. The machines are based on a type of matter known as optical matter in which metal nanoparticles are held together by light rather than the chemical bonds that hold together the atoms that make up typical matter.