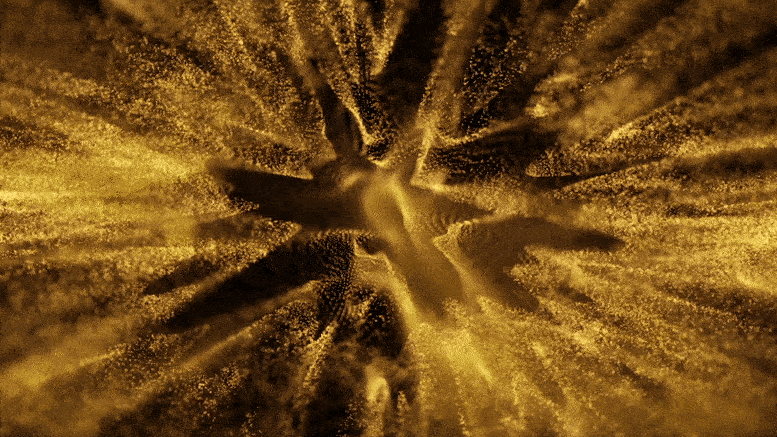
Superconductivity is a phenomenon where an electric circuit loses its resistance and becomes extremely efficient under certain conditions. There are different ways in which this can happen which were thought to be incompatible. For the first time, researchers discover a bridge between two of these methods to achieve superconductivity. This new knowledge could lead to a more general understanding of the phenomena, and one day to applications.
If you’re like most people, there are three states of matter in your everyday life: solid, liquid, and gas. You might be familiar with a fourth state of matter called plasma, which is like a gas that got so hot all its constituent atoms came apart, leaving behind a super hot mess of subatomic particles. But did you know about a so-called fifth state of matter at the complete opposite end of the thermometer? It’s known as a Bose-Einstein condensate (BEC).
“A BEC is a unique state of matter as it is not made from particles, but rather waves,” said Associate Professor Kozo Okazaki from the Institute for Solid State Physics at the University of Tokyo. “As they cool down to near absolute zero, the atoms of certain materials become smeared out over space. This smearing increases until the atoms — now more like waves than particles — overlap, becoming indistinguishable from one another. The resulting matter behaves like it’s one single entity with new properties the preceding solid, liquid or gas states lacked, such as superconduction. Until recently superconducting BECs were purely theoretical, but we have now demonstrated this in the lab with a novel material based on iron and selenium (a nonmetallic element).”